The future
of synthetic
biology
is bio.
Cell-Free fields of application
Synthetic biology is a scientific field which lies at the interface between life sciences and engineering and consists of applying engineering principles namely standardization, modularity, and abstraction to biology. The aim of synthetic biology is to synthesize specific products that respond to particular human needs and its applications go from medicine to agriculture and industrial processes. Through the use of recombinant DNA techniques, synthetic biology designs and develops new biological systems in the laboratory that do not exist in nature, allowing the synthesis of new products with useful applications. Alternatively, it enables the production of already known molecules in a more economical or feasible way.
How it works?
The purpose of a synthetic biology process may be the redesign of a living organism to produce a particular substance that is not naturally synthesized, or the production of a totally new living organism. This can be performed by bacterial gene manipulation, creation of new synthetic metabolic pathways, modification of the DNA molecular structure in order to improve its properties and to allow the synthesis of novel proteins not known in nature, and creation of new regulatory internal circuits in cells that allow to alter cell activity patterns.
Synthetic biology delineates basic bioparts encoded by DNA, which are then combined to produce biochemical reactions. The association of these reactions forms biological pathways that can be grouped together within a cellular unit, which can itself be part of a cellular grouping. Synthetic biology is based on the Design-Build-Test-Learn (DBTL) workflow. The DBTL cycle includes the design of the initial bioparts or the establishment of a preliminary model system to achieve the determined engineering goals, the construction of the bioparts, the testing of their outcomes and the understanding of which engineering strategy is effective and why, as well as the incorporation of the learned knowledge into the decision of the subsequent DBTL cycle (Figure 1).
Figure 1 – The design-build-test-learn (DBTL) cycle. Reproduced from [1].
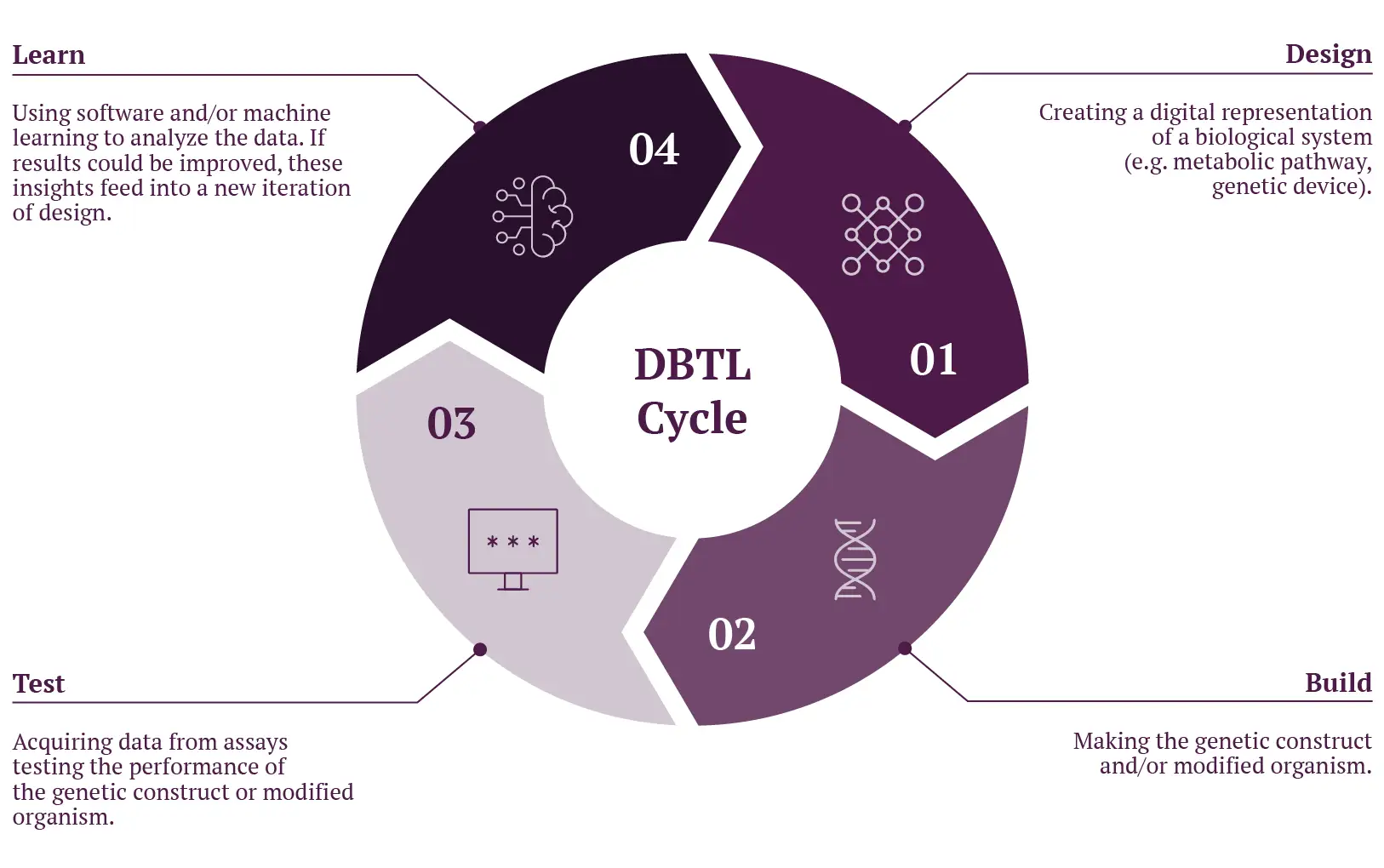
Importance and applications
Synthetic biology allows us to improve our understanding of the mechanisms involved in biology. This technology represents a new way to study living organisms and find out how they work. Since synthetic systems can be much simpler than their natural counterparts, they allow researchers to perform experiments that would be otherwise difficult to carry out or to interpret. But the main benefit of synthetic biology resides on its ability to create new products with social and commercial potential in healthcare, industry and environment (Figure 2).
Synthetic biology allows us to improve our understanding of the mechanisms involved in biology. This technology represents a new way to study living organisms and find out how they work. Since synthetic systems can be much simpler than their natural counterparts, they allow researchers to perform experiments that would be otherwise difficult to carry out or to interpret. But the main benefit of synthetic biology resides on its ability to create new products with social and commercial potential in healthcare, industry and environment (Figure 2).
Figure 2 – Overview of synthetic biology including the areas of application of its products, the disciplines using synthetic biology approaches and the technologies sustaining them. Reproduced from [2].
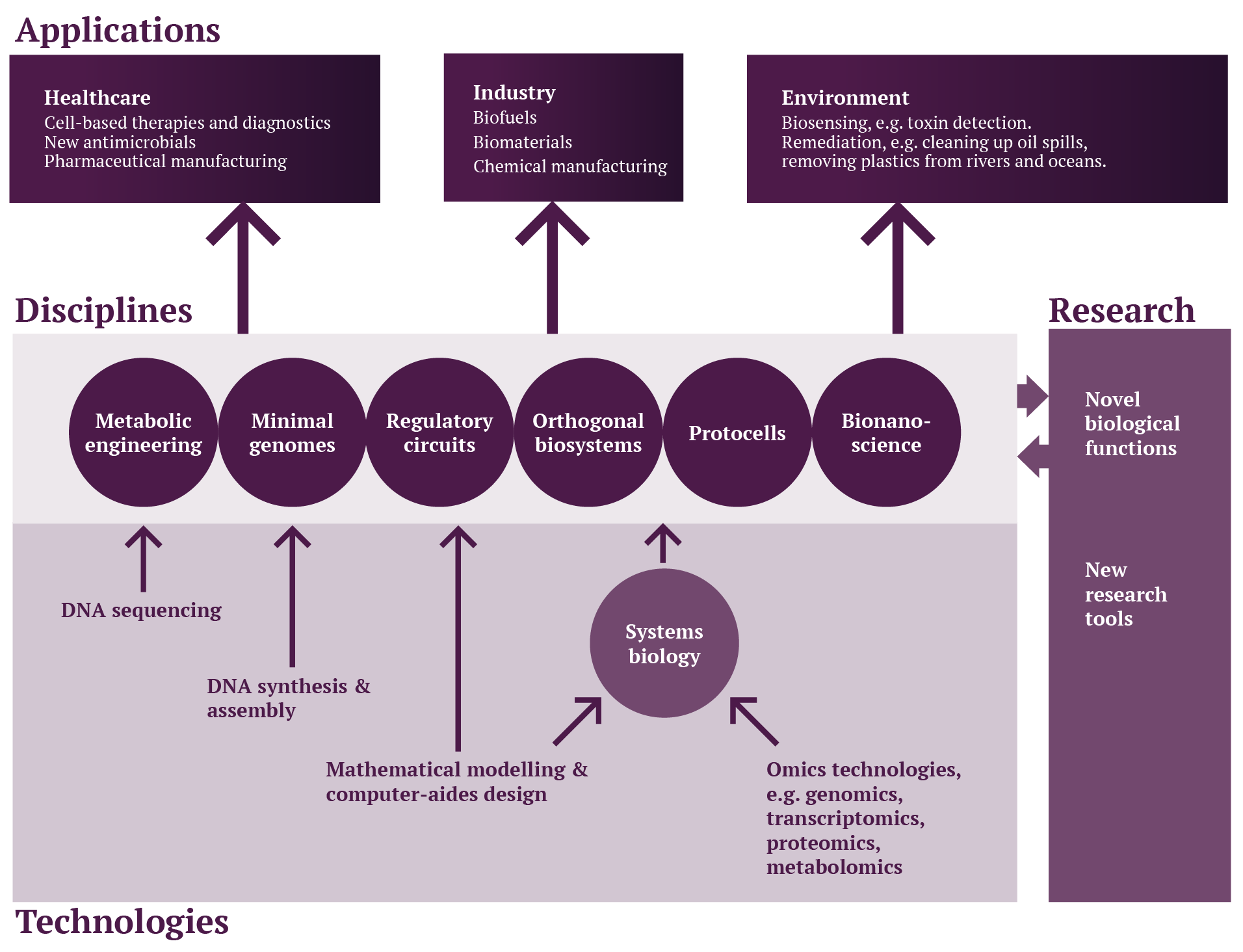
In the medical and pharmaceutical sectors, synthetic biology allows the production of new drugs, vaccines, innovative diagnostic agents and new biologic tissues. For instance, taxol, an anti-cancer agent, is produced by Saccharomyces cerevisiae via synthetic biology technology with yields higher than those obtained when it is extracted from its natural source [3]. Also, the production of artemisinic acid, a precursor of artemisinin (an antimalarial drug), by modified yeast cells or Escherichia coli cells, enables a reduction in its price in around 90% versus the drug traditionally obtained from the plant Artemisia annua [4].
In the chemical sector, synthetic biology allows the synthesis of compounds that are difficult or expensive to produce. Isobutene is an example, serving as a precursor for the manufacture of plastics, rubbers, lubricants and fuels. It can now be produced by a metabolic pathway created by synthetic biology from renewable resources such as agricultural and forestry waste, starch, sugar cane or beet [5].
In the energy sector, microorganisms can be reshaped to produce hydrogen or to perform artificial photosynthesis, or to decompose biomass to produce energy, as an alternative to fossil fuels [6].
In the environmental field, synthetic biology can help in the detection of pollutants and in its degradation or removal, through the use of biosensors [7].
In agriculture, new food additives may be developed using synthetic biology. Additionally, synthetic biology allows the development of increasingly customizable and resilient crops grown without allergenic agents, or the design of new pesticides that are more respectful of the environment and healthcare concerns [8].
Trends and challenges
In the future, synthetic biology will continue to explore metabolic engineering, creating new metabolic synthetic pathways to produce compounds of interest that cannot be naturally synthesized by living organisms, namely new drugs and new biofuels. A different strategy to synthesize new products by cells will be based on the creation of new internal circuits that can change the cell’s activity pattern. It will be possible to create new artificial gene networks that, when introduced in suitable cells, may be used to detect and correct metabolic alterations such as the ones occurring in diabetes, for example.
It is expected that new progress in bioremediation and biodegradation will emerge, in order to assist waste management of environmental pollution. Also, advances in microbiome engineering to address environmental or agricultural concerns are expected. For instance, engineered bacteria for nitrogen-fixation to replace chemical fertilizers, and genetic modification of plant species to induce disease resistance or improved nutritional composition. Finally, we may assist to progress in the technical refinement of novel delivery systems and chemistries to modify organisms, such as the use of modified viruses and nanoparticles, DNA nanotubes or engineered proteins.
Public expectations are high, particularly concerning the production of new drugs and biofuels. Nonetheless, and in spite of the observed improvements, the field has not reached its fully mature phase and depends on further developments in genetic engineering tools. Besides, there are difficulties in standardizing complex biological systems and engineered circuits may not work the same way in different systems, requiring further parameter optimization. Moreover, cells have a limited pool of resources required for biological processes, and the addition of a synthetic biopart represents an extra load on the cell’s activities, leading to slower growth rates and lower protein synthesis rates [2].
Cell-free systems and synthetic biology
Cell-free protein synthesis efficiently addresses the current challenges of synthetic biology. Although E. coli has been used as cellular model in synthetic biology since its beginning, providing the necessary hardware to run the synthetic system, it may be difficult to control and unexpected side reactions can occur.
Cell-free methods have been applied in synthetic biology, by combining the cellular components required to drive the system. These components may be in the form of crude extracts or purified proteins, produced from bacteria or in a cell-free system. Removal of the cell membrane enables the direct access to the inner space of the cell and, thus, a substrate required to a reaction can be easily added to the test tube and the reaction products can also be easily removed and purified. The state of the system can be monitored and rapidly sampled. Furthermore, a cell-free system can be scaled up to allow industrial production.
Recent publications demonstrate the potential of associating cell-free systems to synthetic biology processes [9,10].
Publications
References
To know more
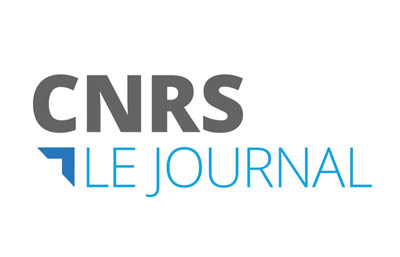
CNRS - The journal
The ABC of synthetic biology

Ministry of Economy - France
Synthetic biology - Achievements
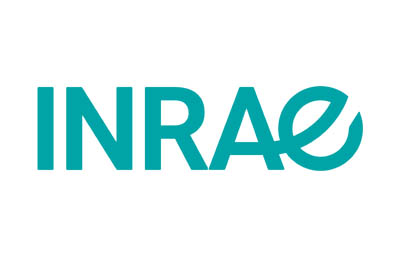
INRAE - (pdf)
Opinion of the Ethics Committee on Synthetic Biology
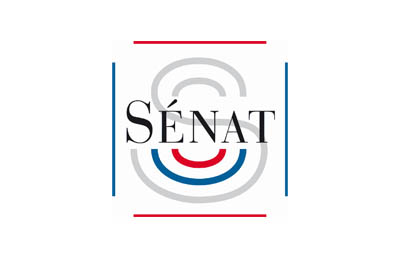
Senat - France
The challenges of synthetic biology
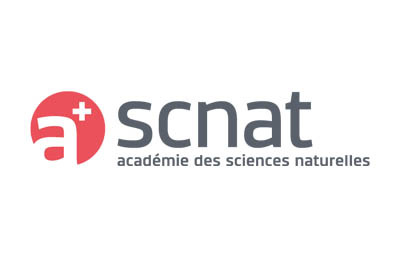
SCNAT - Academy of Natural Sciences
What is synthetic biology?
We look
forward to
your questions
Even
a protein
needs
to express
its potential.
Cell-Free Systems Applications